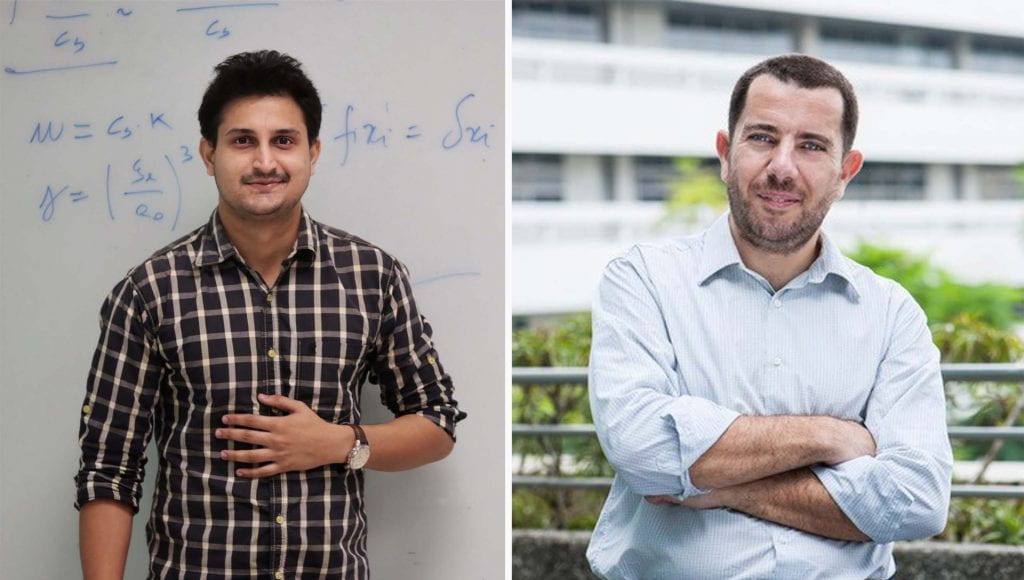
PhD student Shivam Mahajan (left) and Associate Professor Massimo Pica Ciamarra (right) have found an explanation for the anomalous behaviour of sound waves in disordered materials.
In certain materials, such as glass and metal alloys, the atoms are connected in a rigid but irregular fashion. These “disordered solids” behave very differently from crystalline solids like pure copper or salt, whose atoms are arranged in simple repeating patterns such as cubic grids. Despite decades of intensive research, many disordered solids’ properties remain poorly understood because of the difficulty of analysing their irregular atomic arrangements.
Recently, an important advance in the understanding of disordered solids has been achieved by physicists at Nanyang Technological University, Singapore (NTU Singapore). Associate Professor Massimo Pica Ciamarra, a faculty member at the School of Physical and Mathematical Sciences in NTU Singapore, and his PhD student Shivam Mahajan, have managed to explain two anomalous features of vibrations in disordered solids, which had been a longstanding source of disagreement between theory and experiment. Their findings were published in the journal Physical Review Letters in November 2021.
Disordered Solids Flout Theoretical Expectations
Physicists have long been interested in the vibrations of atoms in solid materials, which are responsible for transmitting sound and letting heat flow through solids. In crystalline solids, such vibrations can be described by well-established theories, which do a good job at predicting the resulting acoustic and thermal properties of the materials. However, the situation for disordered solids is more troubled.
The simplest and most widely-used theoretical model for vibrations in solids, introduced by the Dutch-American physicist Peter Debye in 1912, treats the vibrations as plane waves moving freely through the material. It predicts that the number of distinct ways in which an atomic lattice can vibrate at any given frequency – a quantity called the “density of states” – is proportional to the square of the frequency.
Disordered solids, however, do not behave according to Debye’s law. At specific frequencies, they have a substantially higher density of states (i.e., more modes of vibration) than expected, an anomaly known as the “boson peak”.
Moreover, in disordered solids, very low-frequency vibrations comprise the plane wave assumed by Debye’s model, involving the motion of all atoms, and vibrations involving the movement of atoms concentrated in specific random locations. These localised vibrations identify regions that are mechanically softer than the rest of the material.
Many competing theories have attempted to explain these anomalies without definitive success. One difficulty is that the two distinct anomalies – the boson peak and the existence of non-wavelike low frequency vibrations – happen at different frequencies, making it hard to explain both effects in a single theoretical framework.

A disordered solid is formed by atoms, depicted here as small circles, packed in an irregular arrangement. Special regions that are mechanically softer the their surroundings, coloured here in red, give rise to unusual vibrational properties. Figure credit: M. Pica Ciamarra and S. Mahajan