In a recent article reported in the journal Science Advances, a team comprising scientists from NTU’s School of Chemistry, Chemical Engineering and Biotechnology (CCEB) led by Associate Professor Tan Howe-Siang, in collaboration with researchers from other international institutions, reported on their success in mapping the intricate ultrafast energy transfer network involved in the initial step of plant photosynthesis.
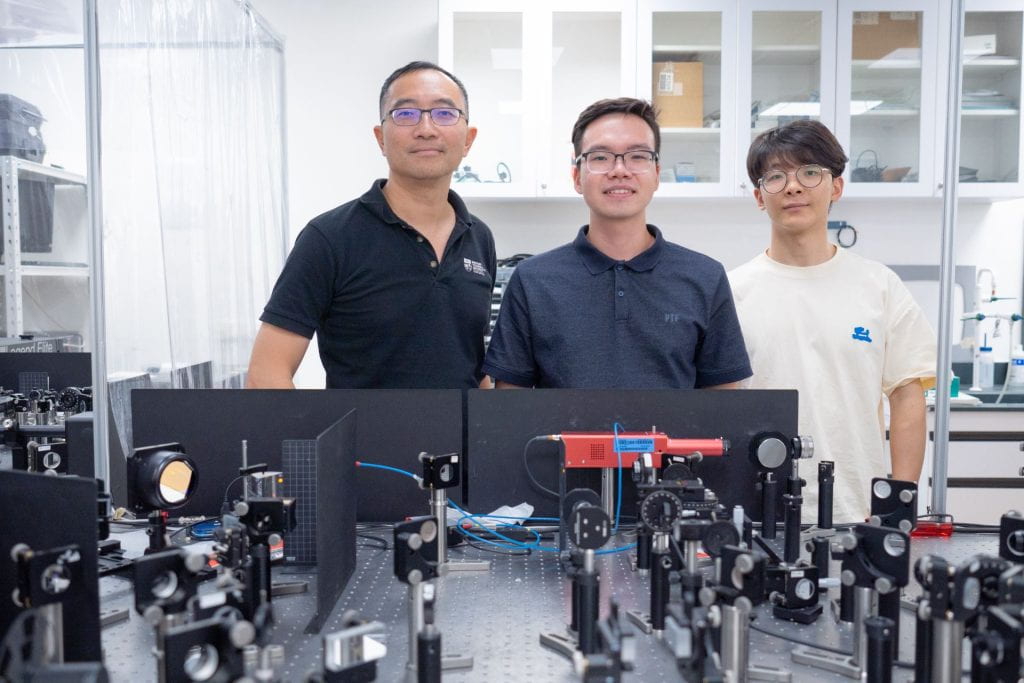
Associate Professor Tan Howe Siang (left), Research Fellow Dr Nguyen Hoang Long (centre) and PhD student Zhong Kai (right) standing in front of the optical table that holds the components of the home-built ultrafast two-dimensional electronic spectrometer in NTU CCEB.
Photosynthesis
Plants rely on photosynthesis to convert light energy from the sun into storable chemical energy, producing oxygen as a by-product. Photosynthesis is, undoubtedly, one of the most important biological processes on Earth, as we rely on the chemical energy (in the form of carbohydrates) for sustenance and oxygen for basic survival.
Despite the importance and the intense scientific attention paid to photosynthesis, there is still much to uncover of this complex biochemical process, for example, regarding how sunlight is harvested and channelled to power the relevant chemical reactions.
What is Photosystem II (PSII)?
Photosystem II (PSII) is a protein “supercomplex” that plays an important part in the light-dependent reactions in photosynthesis. This piece of complex photochemical machinery has the size of a hundred-trillionth of a grain of rice and is packed with hundreds of chlorophyll molecules. These molecules are contained in the PSII “supercomplex” which consists of various pigment-protein complexes, which can generally be divided into two groups: the peripheral light-harvesting antennae complex, and the core complex.
Light energy from the sun is mostly absorbed via chlorophyll molecules, which give plants their green colour. Most of these molecules are generally located in the peripheral light-harvesting antennae complexes, which as the name implies, “harvest” the sunlight. These molecules get “excited” and pass this energy onto neighbouring pigment molecules. The energy gets relayed from antennae complexes to complexes, and eventually reaches the reaction centres in the core complex of PSII, where the photochemical reaction initiates a series of reactions leading to the production of energy molecules, with oxygen as a by-product.
A basic breakdown of the peripheral light-harvesting antennae in PSII
Each PSII supercomplex has about 20 subunits of these peripheral light-harvesting antennae, and each contains approximately 12 to 15 chlorophyll molecules each. Within PSII, there are two layers of energy transfer networks: the intra-subunit network and the inter-subunit network. The intra-subunit network describes energy transfers within each light harvesting antennae, the inter-subunit network describes the energy transfers between each light-harvesting antennae and between the light-harvesting antennae and the core complex.
While the intra-subunit network has been well studied, the sheer number of chlorophyll molecules involved in the inter-subunit network, as well as the difficulty of preparing samples, means that the inter-subunit network remains relatively unexplored.
Understanding the inter-subunit energy transfer networks of PSII
To measure the energy transfer processes involved in PSII, which are in the order of tens of picoseconds (one-trillionth of a second) to tens of femtoseconds (one-quadrillionth of a second), the NTU Singapore team used an advanced ultrafast spectroscopic tool, known as the Ultrafast Two-Dimensional Electronic Spectroscopy technique, or 2DES.

(Above) Ultrafast laser beams impinging on a sample cell that contains a solution of PSII protein supercomplexes, extracted from plants. (Bottom) A typical two-dimensional electronic spectrum of PSII.
Think of the 2DES technique as a camera that has an ultrafast shutter speed that can capture a ‘picture’ of energy transferring in PSII. These ‘pictures’ are spectra spread onto two frequency dimensions, allowing scientists to discern which chlorophyll molecules are ‘connected’ to each other via energy transfer. In 2011, the NTU Singapore team constructed the first 2DES in Asia, now housed at CCEB.
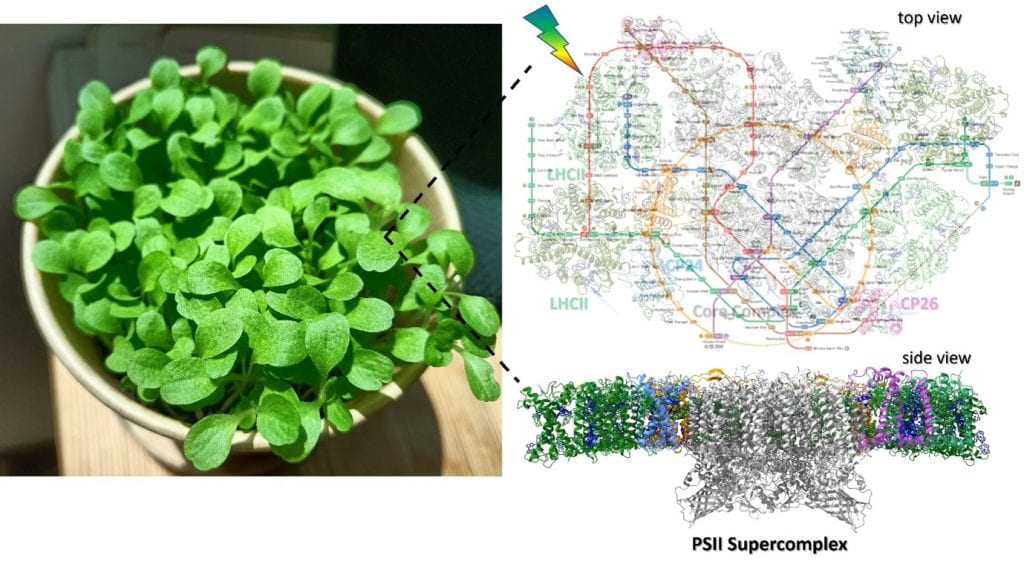
The Photosystem II (PSII) protein supercomplex contains hundreds of chlorophyll molecules (which gives plants its green colour) bundled in separate units of smaller protein units. These units of chlorophyll form a complex network between themselves to relay harvested solar energy to the PSII reaction centre to power the water-splitting reaction. The energy transfer network is like a city railway map and the units of chlorophyll molecules are like the railway stations. The aim of the research is to uncover this photosynthesis energy transfer “railway” network map.
Together with the 2DES measurement data of the various PSII subunits, followed by in-depth analysis and theoretical modelling, the team was able to extract information regarding the inter-subunit energy transfer process. The team describes the whole process of measuring the energy transfer networks in PSII as akin to mapping out the railway network of a city using a special camera in the sky that can only see the trains plying the rail network. By taking loads of photographs at many different times, you can track the movement of the trains. And by piecing together all those photographs, you can eventually build a map of that entire railway network. In this analogy, the tracked trains represent the energy that transfers from chlorophyll molecule to chlorophyll molecule, while the trains stations that are discovered from your mapping are the chlorophyll molecules.
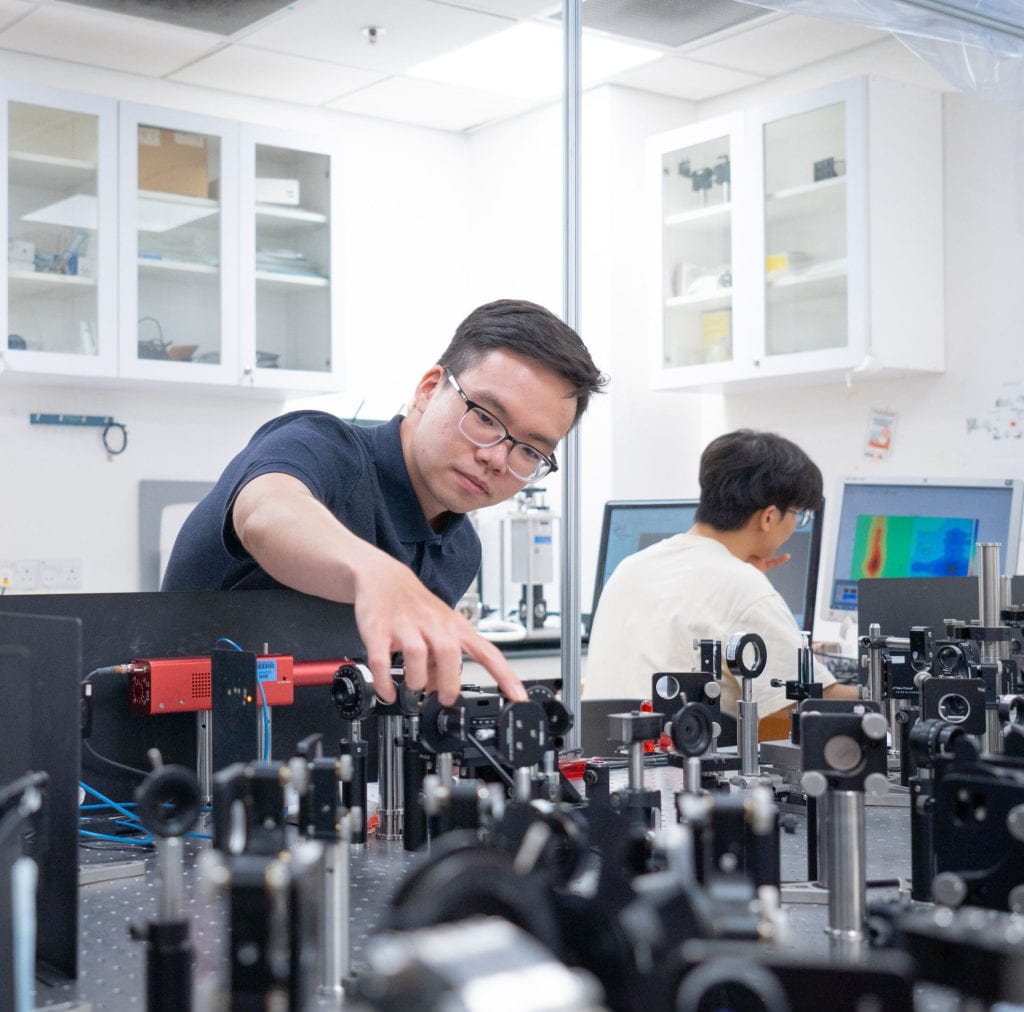
Research Fellow Dr. Nguyen Hoang Long, adjusting the optics in the Ultrafast Two-Dimensional Electronic Spectrometer at NTU CCEB.
Working in conjunction with the NTU 2DES team were researchers from different scientific disciplines, in an international collaborative effort. Plant biologists and biophysicists from the Biological Research Center, in Szeged (Hungary), and Aix Marseille Université (France), helped to grow, extract, and prepare the plant photosystem supercomplexes, as well as created viable models to understand the experimental data. Theoretical and computational chemical physicists from the University of Groningen (The Netherlands), aided in performing high level computer-based simulations to connect hypotheses to experimental data.
What does this mean for the future?
Apart from purely scientific reasons in understanding this most important of biological processes, there are also potential practical benefits. Photosynthesis is amazingly complex but robust. Plants have naturally evolved regulation mechanisms relying on the adjustments in the energy transfer network in the fluctuating light conditions over the normal course of the day. Understanding these networks may help us engineer new generations of crops with specific capabilities to more efficiently harvest light under artificially controlled conditions, thus increasing crop productivity. Furthermore, the lessons learned in natural photosynthesis can be applied to develop photoelectrochemical systems and other artificial, advanced solar utilization technologies, important in powering the renewable energy economy for future global growth and sustainability.