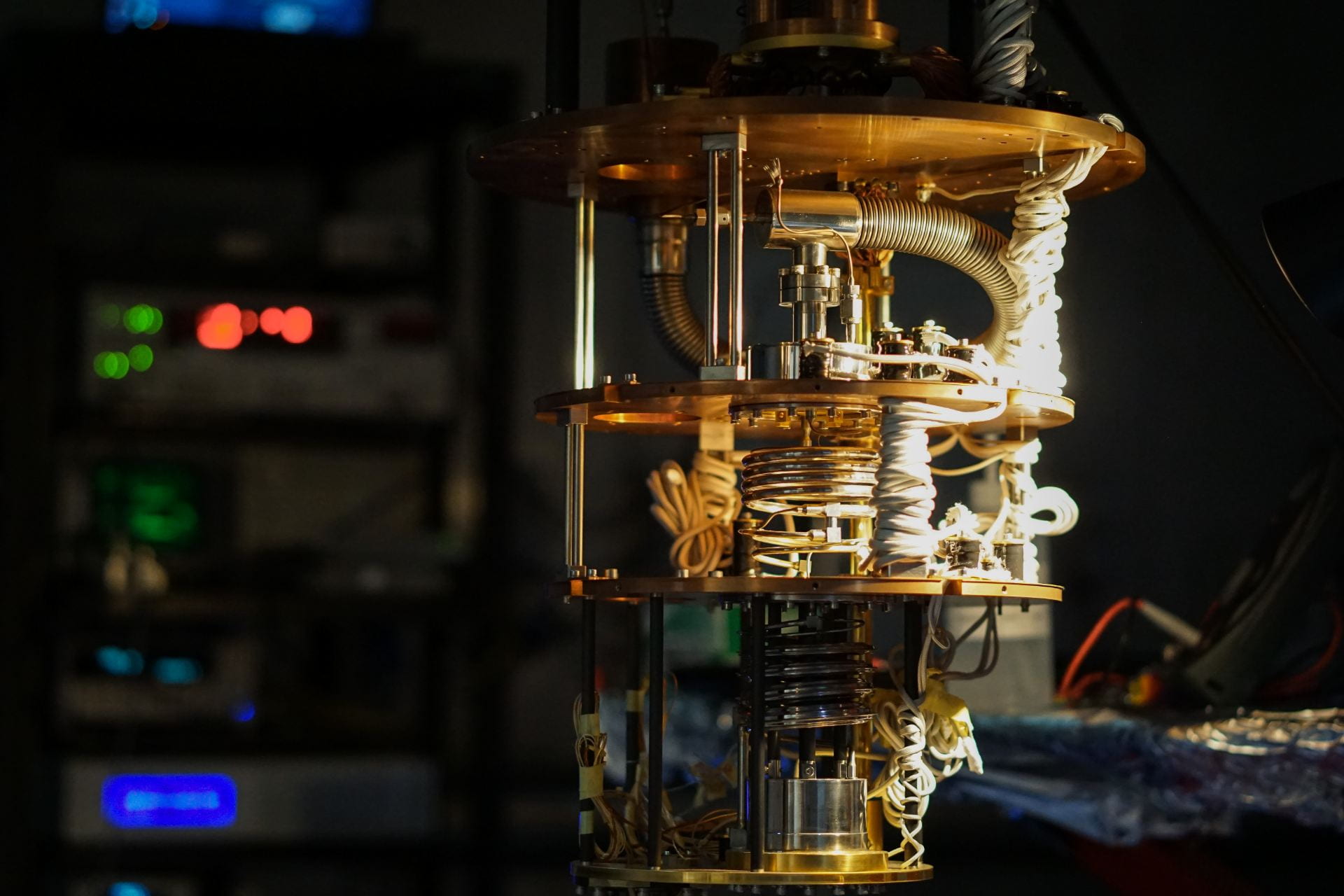
A novel material combining superconductivity with magnetism was developed and studied in Research Professor C. Panagopoulos’ laboratory. The cryostat pictured here maintains the material at ultra-low temperatures while ultra-low-noise electrical measurements are performed on it.
A novel “hybrid” material in which superconductivity and magnetism interact via particle-like entities, known as topological solitons, has been created by an international team of physicists, led by Research Professor Christos Panagopoulos from Nanyang Technological University (NTU Singapore). This breakthrough could form the basis for a new type of quantum computer.
Topological solitons are particle-like phenomena, appearing in certain materials, which are unusually stable against external perturbations. Just as a knot on a string cannot be undone by pulling on the ends of the string, a topological soliton cannot be easily destroyed by disturbing the material. While this may sound like an esoteric concept, various types of topological solitons are known to exist in different forms of matter, from magnets to protein chains. Two types of topological solitons which are of special interest to physicists are superconducting vortices and magnetic skyrmions.
Vortices are topological solitons that appear in certain superconductors – materials that conduct electricity with zero resistance – upon exposure to a magnetic field. They consist of tubes of magnetic field lines, surrounded by nanometre-sized, tornado-like swirls of electric current.
Skyrmions, on the other hand, are topological solitons that appear in some magnetic materials. Ordinary magnets are composed of nanoscopic magnetic “spins” which all point in the same direction. In magnets that have a property known as “chiral instability”, however, the spins prefer to twist relative to their neighbours rather than aligning, and assemble into intricate patterns called skyrmions.
In a paper published in Physical Review Letters in March 2021, Prof. Panagopoulos and his colleagues announced that they have achieved the combination of two different topological solitons – vortices and skyrmions – in a material.
This breakthrough is exciting because theoretical physicists have predicted that combining magnetism and superconductivity in such a way can yield Majorana fermions – exotic particles that act as their own antiparticles. If a superconductor is brought into proximity with a chiral magnet containing skyrmions, the field from the magnet is expected to alter the inter-electron interactions responsible for superconductivity, resulting in the formation of a “topological superconductor” whose vortices contain Majorana fermions.
Majorana fermions have potentially revolutionary implications for quantum computing. In a Majorana-based quantum computer, information can be encoded in pairs of Majorana fermions (i.e., two vortices). Such pairs would be much more resilient to environmental disturbances than the quantum bits used in existing quantum computers.
A major challenge faced by Prof. Panagopoulos and his colleagues was that magnetic vortices and skyrmions do not simultaneously appear, let alone interact, in ordinary materials. To get around this, the team developed a hybrid material combining niobium, a known superconductor, with an atomically-precise magnetic multilayer made of iridium, iron, cobalt and platinum.
“This is a completely new material architecture: a stack of magnets and a superconductor,” says Dr Alexander Petrović, a Senior Research Fellow at NTU who is the first author of the paper. “The carefully-designed properties of this structure allow us to couple the topological solitons from two distinct quantum orders – chiral magnetism and superconductivity – for the very first time.”
The properties of the new material were investigated using many different scientific methods by the researchers, who were based in NTU, Singapore, the University of Geneva in Switzerland, the Technion in Israel, and the University of Antwerp in Belgium. Each of the different analytical techniques was chosen to expose a different aspect of the hybrid behaviour. Electronic snapshots of the superconducting vortices were taken using scanning tunnelling spectroscopy at temperatures a fraction of a degree above absolute zero, while magnetic force microscopy tracked the formation and morphological evolution of skyrmions within the magnetic layer. An extremely sensitive detector known as a Superconducting Quantum Interference Device (SQUID) captured the nucleation of vortices by skyrmions, while the influence of skyrmions on the flux dynamics was deduced from the tiny voltages induced by vortices moving in an applied electrical current.
Computer simulations of the material matched the key experimental results, hence verifying the ability of individual skyrmions to generate vortices in the neighbouring superconductor. These simulations also exposed intriguing flow characteristics for the vortices at high currents, which could enlighten studies of particle dynamics in other complex systems.
“The beauty of this material is that it shows stable vortices above elongated chiral spin textures, as well as isolated skyrmions. This is an ideal geometry in which to attempt manipulation of individual vortices, a prerequisite for computational operations” says Dr. Petrović. “In the future, we aim to explore the electronic properties of our samples for signatures of the anticipated Majorana fermions.”