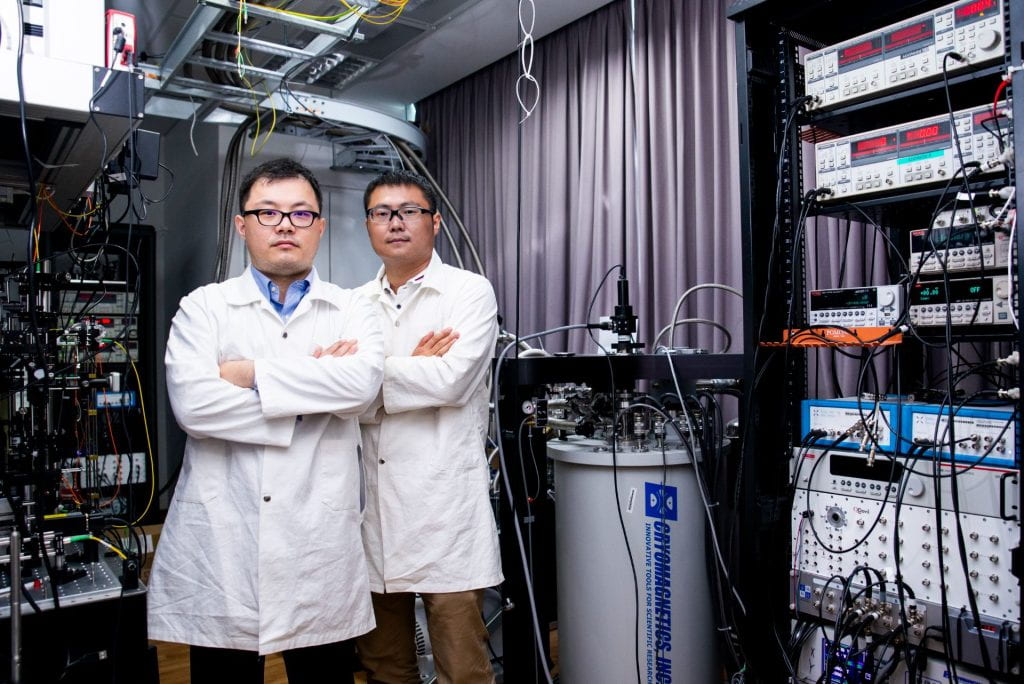
Dr Wang Naizhou (left) and Professor Weibo Gao (right). Their experiment on manganese bismuth telluride, published in Nature in June 2023, may help guide scientists to develop novel quantum materials with unprecedented properties. Photo credit: NTU Singapore.
The surface of the Earth, which we inhabit, is curved. This has many observable effects, like the fact that we can see farther from the top of a building than from the ground. Distances along the Earth’s surface can be misleading—for example, standard world maps misrepresent the relative sizes of the continents by projecting them onto a flat plane. (Antarctica, in particular, seems much larger than it actually is.)
The effects of curvature can also be felt in the microscopic world. In some materials, the motion of electrons is affected by a kind of curvature originating from quantum mechanics. This is not the curvature of a real space, like the Earth’s surface, but rather an abstract mathematical space formed by the set of all possible quantum states of each electron.
Just as the continents’ sizes differ from what is shown on the map, physicists believe that this quantum curvature warps the motion of electrons in materials. Theoretically, this is accounted for via a construct called a “quantum metric”, which quantifies distances within the curved quantum space. Yet in spite of the quantum metric’s conceptual importance, its effects have been too subtle to detect experimentally, until now.
A team led by Professor Weibo Gao from NTU’s School of Physical and Mathematical Sciences has finally uncovered the presence of the quantum metric in a material. By measuring the electrical properties of a material called manganese bismuth telluride (MnBi2Te4), they have obtained the first experimental results that are unambiguously affected by the quantum metric. These findings, which were published in the journal Nature in June 2023, can help guide scientists to develop novel quantum materials with unprecedented properties.
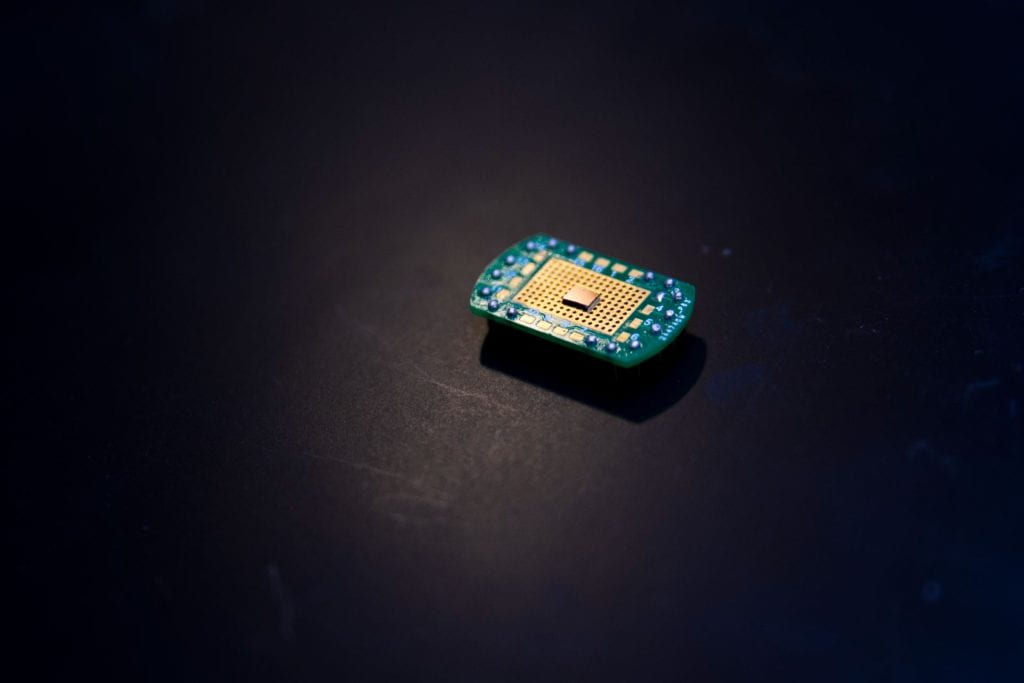
A sample of manganese bismuth telluride, used to perform the first experimental detection of the “quantum metric” of a material. Photo credit: NTU Singapore.
Looking Beyond Ohm’s Law
When an electron experiences a force, it undergoes an acceleration and thereby changes its quantum state. This corresponds to a shift within the space of possible quantum states, and is influenced by the curvature of that space. Therefore, one of the most promising ways to study the quantum metric is to carefully scrutinize the effects of an electric field on the electrically-charged electrons in a material.
Most electrically conducting materials obey Ohm’s Law, which states that the voltage and current are proportional to each other. However, this type of electrical behavior turns out to be unsuitable for detecting the quantum metric, because it is dominated by unrelated phenomena (e.g., how the electrons are deflected by random impurities within the material).
To get around this, Professor Gao and his group turned to a phenomenon called nonlinear transport. At low temperatures, some materials violate Ohm’s law and exhibit a voltage that scales quadratically with the current. In this case, the material’s electrical properties are dominated by quantum effects, including but not limited to the state space curvature described by the quantum metric.
The NTU researchers fabricated a specialized magnetic material called even-layered Manganese Bismuth Telluride (MnBi2Te4). In its ordinary form, MnBi2Te4 is a single layer of atoms (i.e., a 2D material). By stacking together an even number of MnBi2Te4 layers, the researchers created a material whose magnetic properties were fine-tuned to eliminate all other effects that could influence nonlinear transport, aside from the quantum metric.
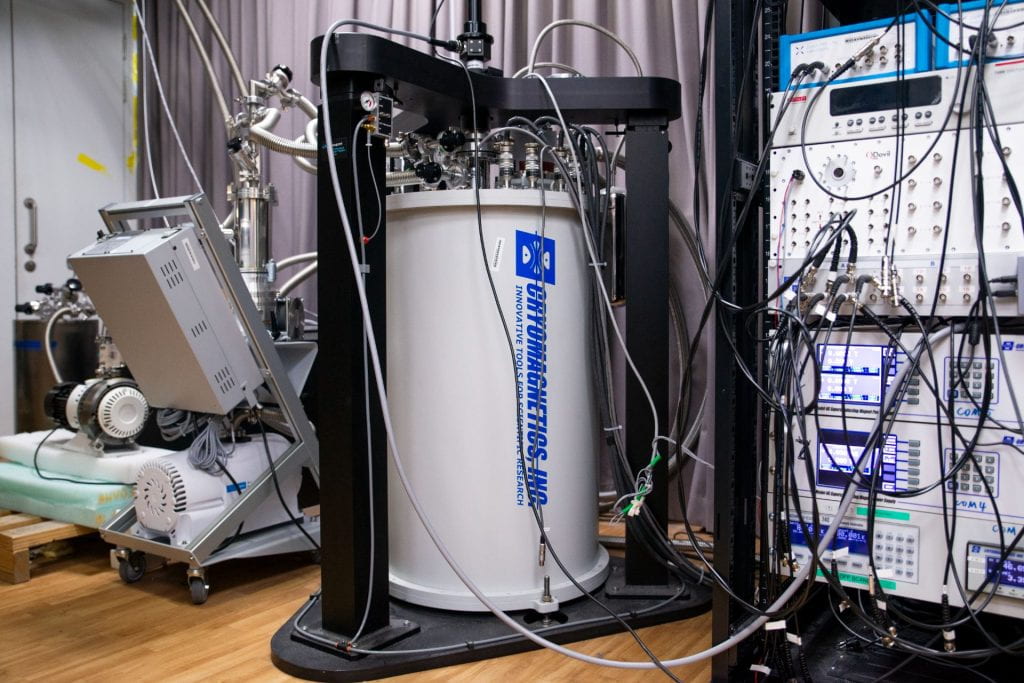
The cryogenic apparatus used by Professor Weibo Gao’s research group to measure nonlinear transport at temperatures close to absolute zero. Photo credit: NTU Singapore.
The Search for a Quantum Signal
The team placed a sample of 4-layered MnBi2Te4 in a cryogenic apparatus, and carefully measured its electrical properties. As expected, they found that the voltage scaled quadratically with the current, showing that the material exhibited nonlinear transport. Strikingly, the nonlinear signal did not depend on the temperature of the material; it scarcely changed as the temperature was raised from 4 K to 10 K (i.e., -269°C to -263°C). This indicated that the nonlinear voltage was an “intrinsic” property of the material, stemming from the quantum features of the electrons.
The researchers observed two other key facts about the nonlinear voltage. First, it varied with the density of electrons present in the material. Second, when the “antiferromagnetic state” was flipped—meaning the internal magnetic fields inside the material switched directions—so did the nonlinear voltage.
To interpret these findings, Professor Gao and his team collaborated with Professor Bing Hai Yan at the Weizmann Institute of Science in Israel. Using theoretical calculations, Professor Yan’s group helped show how the observed variations in the nonlinear voltage could be explained by changes in the quantum metric.
Applications of an Amazing Antiferromagnet
The fact that the nonlinear voltage depends on the material’s antiferromagnetic state is especially significant, as it may have technological applications. The antiferromagnetic state is extremely difficult to determine by conventional methods. By providing a way to “read off” the antiferromagnetic state, the nonlinear voltage measurement may allow antiferromagnetic materials to be used as data storage devices.
“Our discovery provides a straightforward way to read data stored in antiferromagnets by being able to distinguish the two states the materials can take,” explains Professor Gao. “The findings advance research in using antiferromagnets for computer memory in the future.”
The team is currently exploring other antiferromagnetic materials that can encode data at room temperature, using the nonlinear voltage method to “read off” the antiferromagnetic state. Potentially, such devices could store information at much higher densities than present-day computer memory.
Professor Gao’s team is also looking into using materials similar to MnBi2Te4 to make nonlinear electrical components called rectifiers. Present-day rectifiers, based on semiconductor “p-n junctions”, are manufactured by a complicated process of adding chemical impurities to different areas of a chip. In MnBi2Te4, however, the rectifier functionality is easier to achieve since it is a property of the material itself. Such “junctionless rectifiers” could be useful for making certain kinds of low-frequency photodetectors and other devices.